
What Are Magnets?
Have you ever played with metal things that seem to push each other away or pull themselves together (they might have looked something like the bar on the right)? We call these special metal things that can interact with each other without touching magnets, or say that they are magnetic (The University of California 2005)..
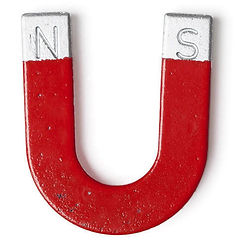
(IndiaMART InterMESH Ltd 2018)
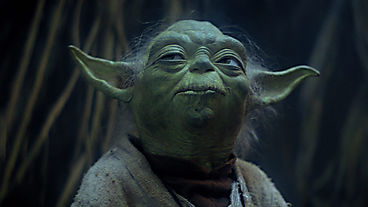
(Baver 2017).
Forces, They make
Magnets are different from other objects because they push and pull each other around using magnetic forces - forces they they 'create' just because they are magnets (Roldán 20100. In year 7, you would have learnt (or may be learning) about forces, the things that make things repel (push apart, or move away from each other) or attract (come closer to each other) (The Australian Curriculum, Assessment and Reporting Authority 2018;The University of Kansas 2013). Forces make things move, hold things together or apart, or change how they are moving (change their direction or speed; The University of Kansas 2013).
Forces are defined as actions that, when unopposed, make objects accelerate (increase or decrease in speed, or change direction; Winokur 2010).
The Magnetic Force
You should know of two main types of forces - contact forces (forces that only 'work' when two things are touching each other; you have to hold and either push or pull a door to open it) and non-contact forces (forces that can occur without contact; the force of gravity pulls you towards earth, even if you jump, it even pulls the moon!) (Winokur 2010). The magnetic force is a non-contact force - it will act between magnetic objects no matter how far away from each other they are!
This might sound odd - when you've played with magnets, they may only seem to push or pull each other when they're close together. This doesn't mean that there isn't a force acting between them, it just means that it's so small that you don't notice its effect on the magnetic objects (Duffy 2011). This is because the strength of the force between two magnetic things (or the strength of the magnetic field) decreases as the distance between them increases (Duffy 2011).
​
This also means that magnetic forces can go 'through' objects - if you get two magnets and put a piece of paper between them, they'll still interact with each other (The University of Illinois at Urbana-Champaign 2012))! You can learn more about this on the Transformers page.

Check out these magnets levitating above each other (the pencil is only there to guide them - they're holding each other up)!
(Innovation Frontier Inc. 2018)
The image on the left proves that the strength of a magnetic field (check out the paragraph below for a definition) increases as the distance from the magnetic object decreases (Roiff 2010). If the strength of the magnetic field remained constant regardless of distance, as more weight (more magnets) are added onto the pencil, the bottom weight would fall to the bench, and only one magnet would be left levitating (Roiff 2010). As the distance between the magnets decreases (but the magnets remain levitating) as more weight is added to them, the magnetic field must be stronger when the weights are closer together (Roiff 2010).
Although we can't create an exact formula for the strength of magnetic fields vs. distance from the magnet, because the 'north and 'south' magnetic fields cancel each other out differently (see Can We Add Charge if you're confused), we know that:
​
Magentic Field Strength ∝ 1/(d^n)
​
where d is the distance in metres from the magnet, and n is generally (but not always) a number between 1 and 3 (Appalacian State University 2018).

(Richardson et al. 2015)
Magnetic poles
How do you know if magnets are going to attract (pull each other together) or repel (push each other apart)? All magnets that we've ever discovered or made have two poles - a north pole and a south pole (Iowa State University 2014). As they are found together, we say that magnets have dipoles ('di' means two) (Iowa State University 2014). Opposite magnetic poles attract each other (south attracts north and north attracts south) and 'like', or the same pole, repel each other (north poles repel other north poles and south poles repel other south poles; Erkal 2002). This is why magnets sometimes flip around before pulling each other together - they need to align their poles correctly so the north and south poles can attract (Erkal 2002).

The putty in this image is made of lots of little magnetic granules that rotate so that their poles are opposite to the poles of the magnetic cube, so they are attracted to the magnetic cube (The University of Wisconsin-Madison 2007). This is why the putty seems to 'eat' the magnetic cube (The University of Wisconsin-Madison 2007).
Magnetic Fields
We know that the magnetic force acts between magnets regardless of how far apart they are, but what do we call this ability or potential of a magnet to exert a force (push or pull) another magnet?
​
We say that every magnet has a magnetic field - this is not something you can see, but is a way of representing the force that a magnet can exert on another magnet, and how this force changes around the magnet (i.e. how the magnet can push or pull other magnets around it; Khan Academy 2018; Erkal 2002). As magnets create forces between each other, a magnet has potential energy that is dependent on how close it is to other magnets, and how 'strong' they and the other magnets are (Nave 2017). Magnetic Fields simply represent this potential energy (Nave 2017). The lines and the arrows on them indicate the direction of the force that the north pole of a magnet (and only the north pole, even though a magnet like this can't exist) would 'feel' when only acted upon by the force of the magnet shown, when on a particular spot on a magnetic field line (Iowa State University 2014). This is why magnetic field lines always point from the north pole to the south pole (Iowa State University 2014). Another way to define their direction is the direction that the north end of a compass needle would point in any particular location around a magnetic (Browne 2016).
​
Magnetic field lines also indicate the 'strength' of the magnetic force at any point around a magnet (Iowa State University 2014). The closer together the magnetic field lines are, the stronger the magnetic force - this is why there is more space between them further from the magnet in the diagram (Iowa State University 2014).
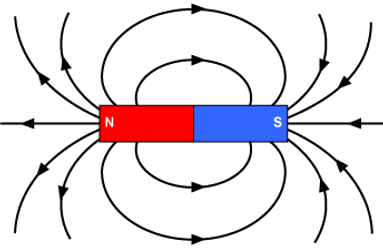
Image of magnetic field lines drawn around a bar magnet (a magnet that takes the shape of a straight bar; North Eastern University 2009)
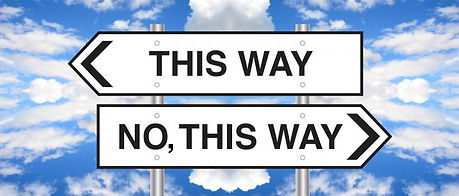
you can't go in two directions at once
(Reginiano 2016)
A bit more on magnetic field lines
-
The magnetic field lines for a particular magnet can't cross:
​
If they could, we'd be saying that a magnet at the location where the field line crossed would go in two different directions - that doesn't make sense (The University of Illinois at Urban-Champaign 2013).

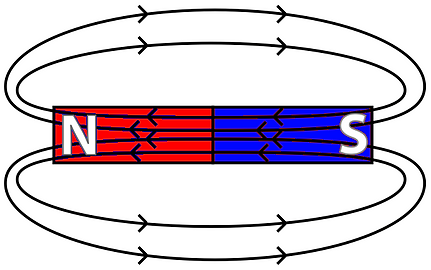
N S
(Silly Beagle Productions 2017)
-
​Magnetic field lines are closed loops, they don't have a beginning or an end:
Yes, that means that they also go through the magnet (Browne 2016). This might seem odd, because unlike poles attract (Browne 2016). But, the image on the left tells us that the north end of a magnet that's inside another magnet would go towards the north of the magnet that it's inside of (Nave 2017). This doesn't really happen, because magnets must exist in dipoles (have both a north and south pole), so we can't really put one magnet inside another, because it would split the poles, which can't happen (Nave 2017). When we put a small magnet inside a big magnet, the magnetic field of the big magnet, in effect, separates into two magnetic fields, because magnetic poles can't exist by themselves (Wagner 2002). You can see this below.​
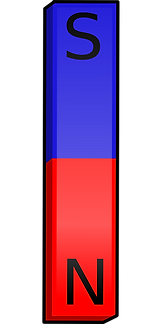
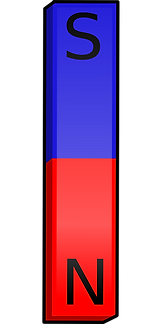
Imagine we put a compass inside the big magnet (the dark it)
Because north and south poles can't exist by themselves, the big magnet 'splits' into two magnets, with their own dipoles
The 'big' magnet doesn't physically split into two magnets, but the magnetic field inside it changes into two different field, each with their own pair of poles (Wagner 2002).
After the magnetic field of the 'big magnet' has been split into two, opposite poles attract and the north end of the compass needle is attracted to the 'north' end of the bog magnet (Wagner 2002). This is why field lines inside magnets point towards their north poles (Wagner 2002).

A quick note for nerds on the direction of magnetic fields
Images: (Pixabay 2018; Roblox Corporation 2018)
This is an image of magnetic field line (image: James Crispin 2018).
The direction of a magnetic field line will show the path that the north pole of a magnet would take, if isolated (even though this can't exist, we call this a north magnetic monopole), when only affected by the magnetic force of the magnet creating the field lines, if it was placed on the field lines and was initially stationary (Browne 2016). In the real world, this can't happen because everything is being acted upon by multiple forces (Browne 2016). So, the direction of magnetic field lines in any particular location represents the direction of the magnetic force that would be exerted on the north pole of a magnet (Browne 2016). This means that, if the north pole of a magnet was 'on' a field line of another magnet, it would 'feel' a force that would push it in the direction of the tangent to the field line (the grey line in the diagram), or in the direction of the field line at that particular point (The University of California, Davis 2018).
Are There Different Types of Magnets?
Yes! There are two main types of magnets, permanent magnets and temporary magnets.
​
Permanent magnets are magnets, or objects, that stay magnetised (keep a magnetic field) even when they are taken out of a magnetic field (Kobes et al. 1999). We call these magnets hard magnets (Livingston 2005). All permanent magnets need to be initially magnetised by another strong magnet (this determines where the poles are on the magnet); its also possible for permanent magnets to lose their permanent magnetism if they heated above certain temperatures (Kobes et al. 1999). We call this temperature, which is different for each magnetic material, the curie temperature.
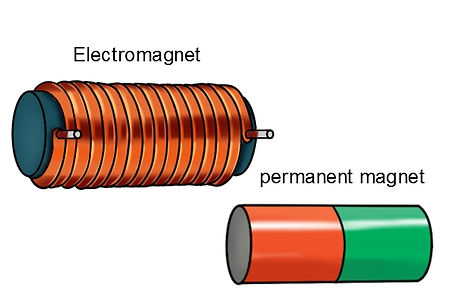
(Jobmaster Magnets Canada Inc. 2017)
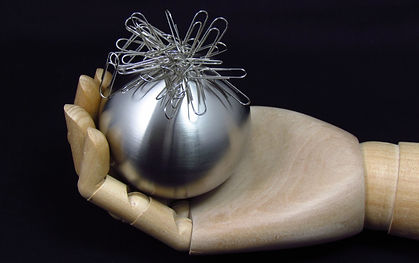
Temporary magnets, that don't require electricity, are magnets that only behave like magnets (only 'produce' a magnetic field) when they are in the magnetic field of another, generally stronger, magnet (Kobes et al. 1999). We say that such magnets are soft magnets (Livingston 2005). However, there is another type of temporary magnet called an electromagnet, which only produces a magnetic field when electricity is flowing through it (Stanford University 2017). Check out how electromagnets work on The 'Electro' Part page!​
Most paperclips are not permanently magnetic, but are attracted to magnetic objects. This is because they are temporary magnets. Image: OfficeSupplyGeek 2018).