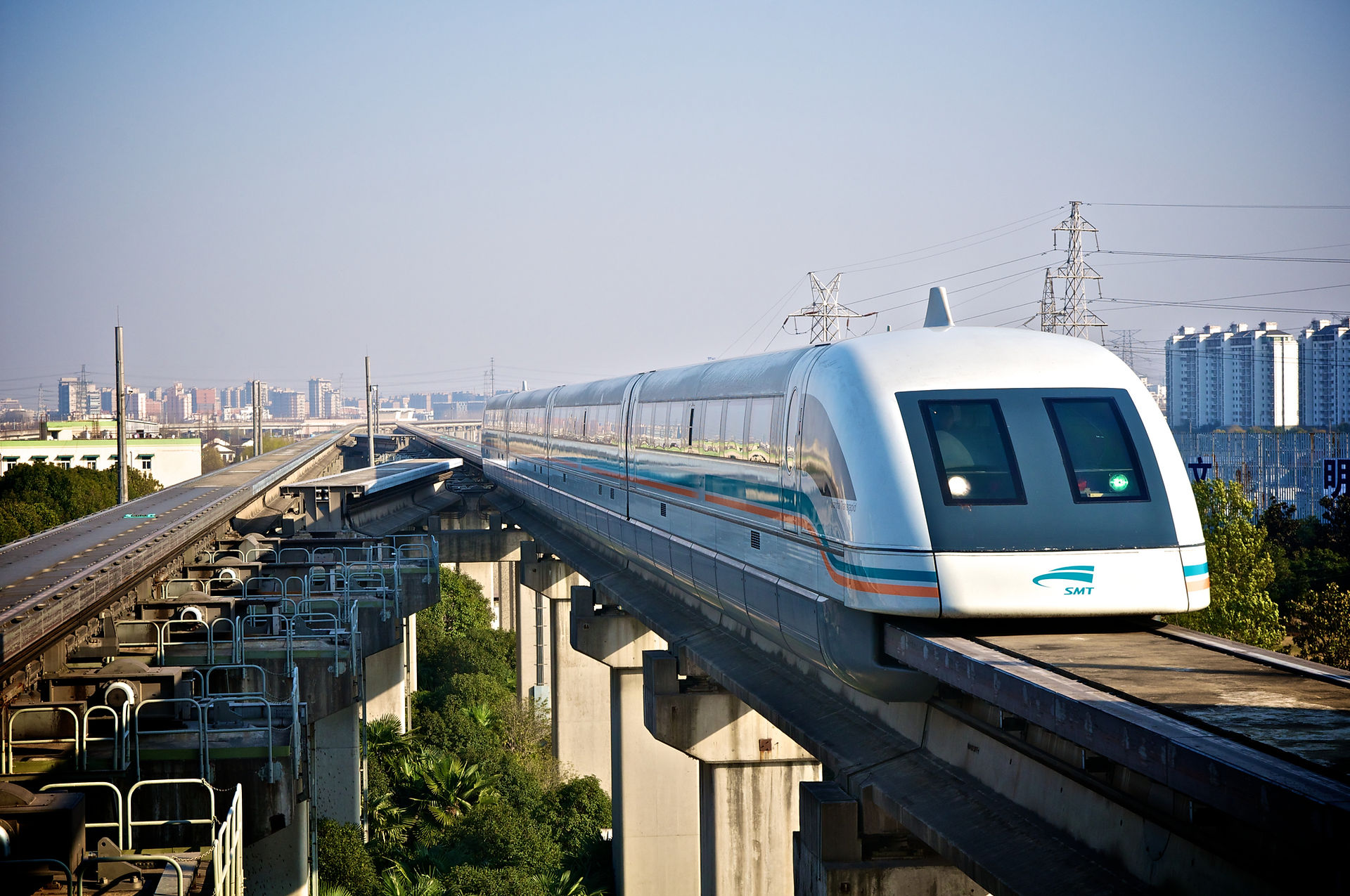
Maglev Trains
Maglev, short for magletic levitation, refers to a special type of train that doesn't need wheels (The United States Government 2016). Maglev trains use magnetism and electromagnets to levitate and move above tracks, without actually touching them (The United States Government 2016)! As they don't have many moving parts (because they don't have wheels or engines), there isn't any friction between the train and the track (The United States Government 2016). This means that they can achive speeds over 600 km/h (Credito 2017)!
What do Maglev Trains Need to Work?
1. Levitation
Obviously, if we don't want the train grinding itself on the tracks, something needs to hold it up (The United States Government 2016). As Maglevs don't have wheels, they need to use magnets to levitate above the track (The United States Government 2016).
Have you ever tried making one magnet levitate above another, by aligning their like poles? Generally, the 'levitating' magnet will be pushed to one side and fall down (Banerjee et al. 2002). If a Maglev train was pushed off its tracks and fell down, it would make a very big, very expensive mess that could hurt people. Maglev trains need something to guide them so that they stay above the track at the right height (Presson 2006).
2. Guidance
3. Propulsion
If we make a train carriage levitate above a track stably, we have made a magnetically levitated room. If we want it to be a train, it needs to move - this means it needs propulsion, or something to move it (The United States Government 2016). Normal trains use engines (like the ones in most cars) or electric motors (motors that use electricity to make things, like the blade in a blender, turn) to turn their wheels, which, using the force of friction, push them along (Harley-Trochimczyk 2009). As Maglev trains don't have wheels or even touch the track, magnets must be used to propel (move) them (The United States Government 2016).
How do Maglev Trains Levitate?
The thing that makes a Maglev train levitate is called its suspension, because it suspends it in the air (Presson 2006). There are Three main types of Maglev suspension: Electromagnetic Suspension (EMS), Electrodynamic Suspension (EDS) and Inductrack (Presson 2006; Letts 2012).

Types of Magnetic Levitation (Letts 2012).
Electromagnetic Suspension

Electromagnetic Suspension Diagram (Presler 2009).
Electromagnetic suspension is based on the basic principle that opposite-poles attract (Wilson 2015). Maglev carriages are built so that they rap around a T-shaped track (check out the diagram), which can be made of a permanent magnetic substance, or can have electromagnets in it (Wilson 2015). Regardless of if the track is made of permanent magnets, electromagnets, or both, an electromagnet that faces upwards and is of the opposite polarity to the 'track magnets' is put inside the bit of the train that raps around the tracks (Wilson 2015). The force of attraction between the electromagnets on the train's undercarriage (the lowest big of the train) and the magnets on the track (whether permanent or electromagnets) pulls the train upwards so that it levitates at about 1 to 10 cm above the track (Presson 2006).
What Happens If The Train Gets Heavier?
As there's always an electromagnet on the train, which is lifting it up, if the train gets heavier, the electrons (which are alternating in direction) can be made to move faster in the wire-coil electromagnets, creating a stronger magnetic field, which pulls the train up with a larger force that counteracts the increase in weight (Wilson 2015). Sensors that detect how high high the carriages are above the ground send information to an on-board computer that changes the electric current in the electromagnets to keep the train at a consistent height above the ground (Wilson 2015). This gathering and exchange of information needs to happen very quickly, as weight isn't the only force acting to push the train down (Massachusetts Institute of Technology 2001). Wind, rain and forces of air resistance can act to push the trains up or down, so the strength of the electromagnets needs to be constantly changed so that Maglevs don't collide with their tracks (Massachusetts Institute of Technology 2001).
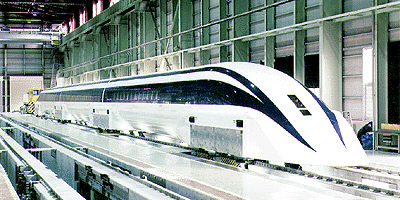
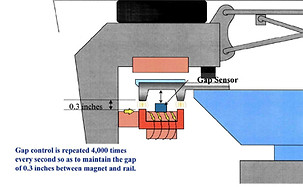
Maglev Gap Sensor Diagram (Latino 2009).
Electrodynamic Suspension
Maglevs that use electrodynamic suspension use the principle that like-poles repel, and special magnets called superconductors to levitate above tracks (Wilcon 2015).
MLX01 Experimental Japanese Maglev Train uses Electrodynamic Suspension (Shinagawa 1997).
What are Superconductors?
All conductors, at room temperature, oppose the flow of electric current - this phenomenon is called electrical resistance (electrons; Travis 1999). When conductors are cooled to really low temperatures, they no longer have any resistance to the flow of electricity, so we call the superconductors - because they conduct without any resistance (Travis 1999). The temperature at which a conductor becomes a superconductor is called the critical temperature, and is different for different materials (Travis 1999). There are two types of superconductors, Type I Superconductors and Type II Superconductors (Nave 2017).
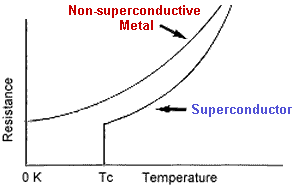
Superconductor Electrical resistance vs. Time Graph (Barry 2003).
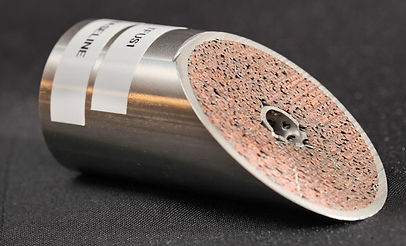
Sample of a Type II superconducting wire (Bardoel 2012).
Type I superconductors are pure materials (they are only made of one 'type' of atom), while Type II superconductors are made of combinations of materials (most are metal alloys; Nave 2017). The biggest and most important different between the two types of superconductors is that type I superconductors only have one, very low critical temperature, while type II superconductors have two critical temperatures that are much higher (The Open University 2018). Type II superconductors can never completely work as superconductors, because they'll always have sections within them that won't act as superconductors (The Open University 2018). Below their lowest critical temperature, these sections will only be around their edges (The Open University 2016). However, when they are between their two critical temperatures, they have much larger sections called vortexes that don't super-conduct (The Open University 2016). Even though the critical temperatures of type II superconductors is low and can only reach about -120°C (some can reach slightly higher temperatures and remain superconducting, but require extremely high pressures), they can be constantly cooled using liquid nitrogen for use in modern technology (Steele 2010).
How do Superconductors Relate to Levitation?
Another cool property of superconductors is that they actively exclude external magnetic fields from them - this is called the Meissner effect (Nave 2017). This mean that if you bring a magnet close to a superconductor, you'll feel a force pushing back on the magnet because the superconductor will create a magnetic field that is equal in strength, but opposite in direction to the magnetic field of the magnet that you are holding (Nave 2017). So, superconductors will repel other magnets around them (Nave 2017). If a superconductor was dropped just above a big magnet, it would create a magnetic field that would repel the magnet below it (Wilson 2015). Unlike trying to align to like-poles of different magnets to try and get one to levitate, superconductors will change their magnetic fields to perfectly oppose any other magnetic fields, so they can't be pushed to one side, and must levitate (Nave 2015).
Another reason why type II superconductors are better to use than type I superconductors is that they have higher critical magnetic fields (Nave 2017). All superconductors can only act as superconductors in certain magnetic fields - if a magnetic field exceeds a certain critical strength, the superconductor will stop acting as a superconductor, regardless of its temperature (Nave 2017)
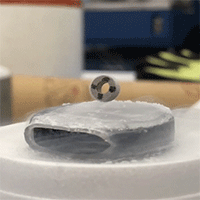
Magnet Hovering Above a Superconductor (The University of Pennsylvania 2018).
But Why?
We call an object that gets a magnetic field induced in it that opposite in direction to an external magnetic field diamagnetic (The University of Minnesota 2002). Lenz's Law and the conservation of energy tell us that if a magnet induces a magnetic field in something else, the induced magnetic field must oppose the magnetic field of the magnet. As a consequence, everything (even material that we say are non-magnetic) are partly diamagnetic - this effect is very small in most materials, so we generally can't detect it without specialised equipment (The University of Minesota 2002). In superconducting materials, nothing stops the movement of electrons, so this effect is very strong and noticeable (The University of Minesota 2002). However, we still don't properly know how the Meissner Effect works (Nave 2017).

Diagram of the direction of a magnetic field induced in a solely diamagnetic material (Sanos 2015)
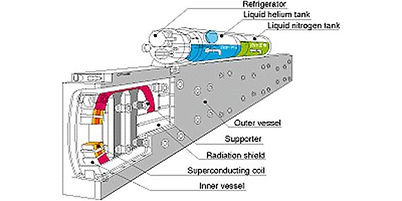
Diagram of the insides of a maglev train that uses EDS (Presson 2006). Note that a magnetic radiation shield is needed to protect the occupants from the strong magnetic fields created by the superconducting coils (Presson 2006).
How Are Superconductors Used in Maglev Trains
As superconductors don't have any electrical resistance, electric currents (charge) can just keep flowing round and round superconductor circuits, with not much energy input (Grace 2014). So, in maglev trains with electrodynamic suspension, a large current, that isn't alternating, is 'put' through superconducting coils, which are cooled using liquid helium and liquid nitrogen (you may have seen a science demonstration using liquid nitrogen - it's very cold; Wilson 2015). This current, (because it is made of moving charge) creates a strong magnetic field (Wilson 2015).
In the track below, there are wire loops that form coils with no power source (Kuhu 2015). As the train moves, the magnetic field of the superconducting coils in the train induce currents in the wire coils in the track, which, because of Lenz's Law, create magnetic field that oppose the train's magnetic field (created by superconducting coils; Kuhu 2015). This makes the train levitate (The United States Government 2016)! However, because the train's superconductor magnetic field is only moving (and changing relative to track) when the train is moving, it can only induce a current in the wire coils when the train is moving (Wilson 2015). This means that maglevs that use electrodynamic suspension need to have rubber wheels to support them, because they need to be at certain speeds before they can levitate (Kuhu 2015).
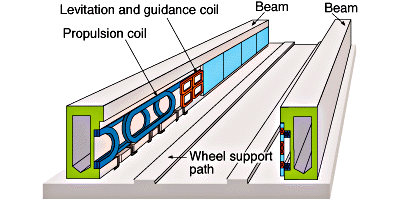
EDS Maglev Track Diagram (Credito 2017).
Inductrack Suspension
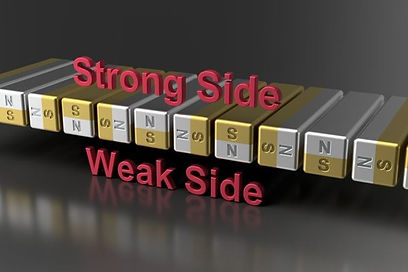
Halbach Array Diagram (Laimer 2017).
Inductrack levitation works on the same principles as electrodynamic suspension, except the superconducting coils are replaced with special permanent magnets, that don't need cooling or an electricity supply (Heller 1998). Normally, permagnet magnets would not be strong enough to allow a maglev train to levitate, but Inductrack suspension systems connect them together is special arrangements called Halbach Arrays (Heller 1998). These have a really strong magnetic field on one side, and almost no magnetic field on the other - so Inductrack maglevs also don't need magnetic shielding (Heller 1998). Looped coils, like the ones used in electrodynamic suspension, are in the track and get currents induced in them when the train moves, creating magnetic field that repel the train from the track, making it levitate (Heller 1998). As magnet fields are strongest closest to their source, if more weight is added to the train, it might ride a bit lower, but still won't touch the tracks (Heller 1998). Like EDS, the magnetic fields produced by the wires in the tracks will perfectly repel the train, keeping it stable (Heller 1998). Inductrack maglevs also need wheels to get up to a speed that allows them to levitate (Heller 1998).
Guidance
All maglev trains use the principle that like-poles repel in their guidance system (Presson 2006). In EDS and Inductrack maglev trains, extra coils are placed up the sides of the tracks - the coils both repel the permanent magnets (inductrack) or superconductive coils (EDS) in the train, keeping the carriages centered (Brown et al. 2011). In electromagnetic suspension system, electromagnetic coils that create magnetic fields opposing the magnetic field of the guide-way (track) are put in the sides of the train (check out the diagram), and sensors are used to detect if the train is centered on the guideway (Brown et al. 2011). If not, the electromagnets in the sides of the train are used to push the train back into the right position - this happens lots of times in a second (Brown et al. 2011).

Image: (Wilson 2015).
EMS EDS and Inductrack
Propulsion
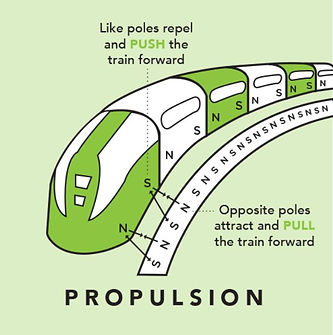
Maglev Propulsion Diagram (The United States Government 2016).
Maglevs use the attractive forces of opposite-poles to pull their carriages along, and the repulsive forces of like-poles to push them (Dona et al. 2017). Most maglevs use a system called Linear Synchronous Motor propulsion (Dona et al. 2017). Earlier, you saw that maglev tracks have propulsion coils in them which act as electromagnets when current is put through them (Dona et al. 2017). These coils are made so they can either push or pull the magnets (or electromagnets) on the carriages (Dona et al. 2017). Computers control them so they switch directions really quickly, so that the coils in-front of the magnets on the train pull the train towards them, and the coils behind the train push it forward (Dona et al. 2017). How quickly these electromagnets change their direction determines the speed of the train (Dona et al. 2017). To stop, the current in the propulsion coils in the track is reversed so that is goes in the opposite direction, and the magnetic fields push the train backwards, slowing it down.
Another way (that isn't used much) uses electromagnets in the train and Lenz's Law to induce electric currents in the track that have magnetic field that repel the train, pushing it forward - this generally uses more energy and has a speed limit, because of the equipment it uses (Dona et al. 2017).